Abstract
Have you ever heard or read about GMO? GMO stands for genetically modified organisms. Scientists can deliberately modify the DNA of organisms, such as bacteria or plants, to change their properties for a specific purpose. For example, crops can be modified to become more drought- or pest-resistant. Genetic engineering is a very powerful tool in biotechnology that has already found many different applications in agriculture, medicine, and industry. In this project, you will engineer a non-hazardous strain of Escherichia coli bacteria yourself by inserting a fluorescent protein gene (GFP) into their DNA. If you are successful, you will make your bacteria glow under UV light!Summary
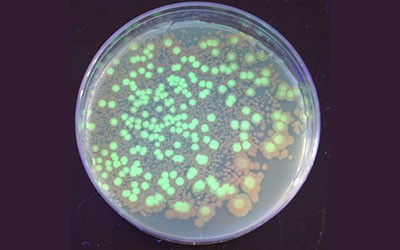
Objective
To determine transformation efficiencies dependent on transformation conditions, while genetically modifying Escherichia coli bacteria so they glow under UV light.
Introduction
Imagine if you could change the appearance or function of any living organism in the world. You could create a cat with pink fur or make a tree that grows candy. The possibilities are endless! This is not as impossible as you might think. Scientists have already figured out how to change the genetic code of living organisms. This process is called gene engineering, gene modification, or gene manipulation. You probably know that all living organisms have DNA in their cells that contain the genetic information for how they develop and function. So how can you make changes to this genetic information?
Genetic engineering uses biotechnology to alter the genes of an organism. A gene is a specific DNA sequence that encodes the synthesis of a protein. When a gene is expressed within an organism, the DNA is first copied into RNA in a process called transcription. The RNA is then converted into a protein during another process called translation (Figure 1). Proteins are like little nanomachines that carry out most functions inside a cell. In a human organism, for example, they help digest our food, keep our heart beating, and control how our muscles move.
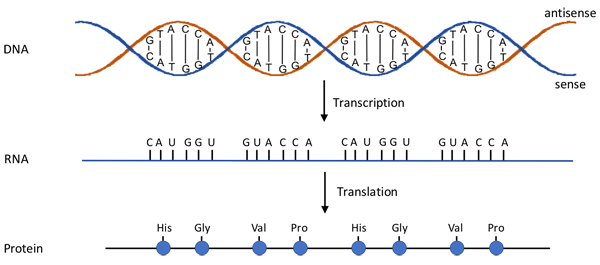
Figure 1. A DNA sequence is first transcribed into RNA, and then translated into a protein.
The key to changing an organism's property or function is thus to alter its genes. You can either modify or delete an existing gene within an organism or you can add a new gene into its DNA. Creating a genetically modified organism (GMO) involves multiple techniques and several steps. Let's have closer look at how bacteria cells can be genetically modified. First, genetic engineers need to choose the gene that they want to modify, insert, or delete. In the next step, all the DNA fragments necessary for the modification of the bacteria need to be created and introduced into the host cell. The host organism is the bacteria to be modified. DNA molecules that are formed by laboratory methods to create new DNA sequences are called recombinant DNA.
The technique that allows us to create and assemble recombinant DNA molecules is called molecular cloning (Figure 2). New or altered DNA sequences can either be synthesized or created by using one of several cloning methods. One of these methods includes cutting and pasting existing DNA pieces together. To cut existing DNA fragments, restriction enzymes are often used, which are able to break apart the DNA at a specific site. Once broken apart, new or altered DNA fragments, or specific genes of interest, can be inserted. The enzyme DNA ligase is then used to fuse the individual DNA fragments of the recombinant DNA together. The next step is to introduce the newly created recombinant DNA into the bacteria cell. In molecular cloning, this is usually done with a vector. Vectors are DNA molecules that carry recombinant DNA into the host cell. Once inside the host cell, the introduced DNA can then be replicated and/or expressed. The most commonly used vectors are plasmids (Figure 2). Plasmids are circular DNA molecules that naturally occur in bacteria. They are usually small, separate from the chromosomal DNA of the cell, and often carry genes that provide a benefit for the bacteria, such as antibiotic resistance. For molecular cloning, artificial plasmids are created and the target gene or DNA sequence is incorporated into the plasmid DNA. This way the whole plasmid, including the recombinant DNA, can be introduced into the bacteria host cell.
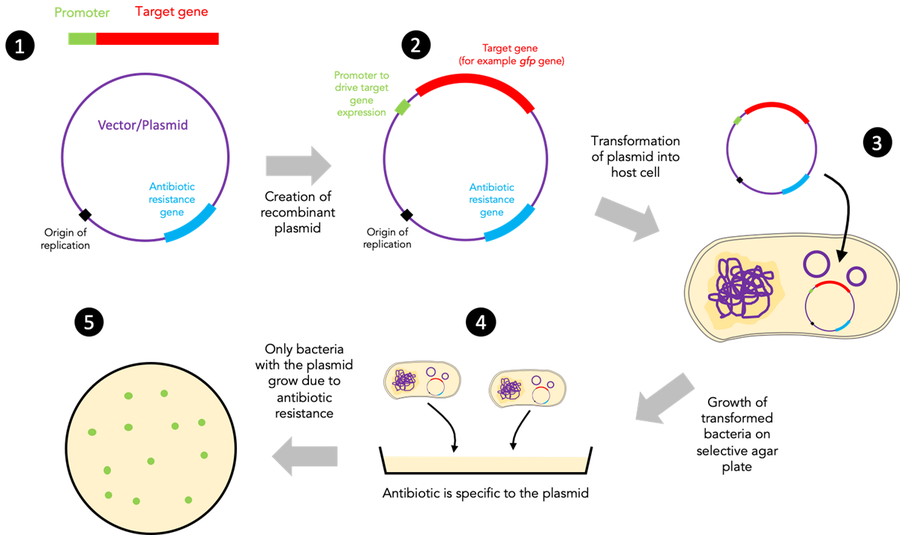
The first step shows a circular plasmid that contains an antibiotic resistance gene and a separate linear target gene. The second step shows the recombinant plasmid, which has the target gene incorporated in its circular structure. The third step shows the plasmid with an arrow pointing to a schematic drawing of a bacteria cell. The bacteria cell contains chromosomal DNA, as well as three circular plasmids with one of the plasmids being the plasmid with the target gene. The fourth step shows a schematic drawing of a selective agar plate. Two bacteria cells that include the target gene plasmids are shown above the cells with arrows that point toward the agar plate. The fifth and last step shows a top view of an agar plate with many circles on it that represent individual cell colonies.
Figure 2. Schematic drawing showing some of the steps involved in generating genetically modified bacteria.
The actual modification of the bacteria's genetic material happens when the recombinant DNA is integrated into the bacteria cell. The uptake of the plasmid into the bacteria cell is called transformation. In the laboratory, transformation can be triggered using multiple methods, such as chemical treatment of the bacteria cells or electroporation. Electroporation uses high-voltage pulses to increase the permeability of the bacteria cell membranes, which allows foreign DNA to enter the cell. In bacteria, plasmids can also be transferred from one cell to another via direct cell-to-cell contact. This process is called conjugation. During transformation or conjugation, not every bacteria cell will take up the plasmid, which is why the successfully transformed bacteria have to be distinguished from the unsuccessful ones. For that purpose, the plasmids used for molecular cloning usually contain an additional selective marker besides the target gene or altered DNA sequence. This marker, which is often an antibiotic resistance gene, allows us to selectively grow the genetically modified bacteria on agar plates that contain the specific antibiotics. In many cases parts of the plasmid DNA of a successful transformant is sequenced to confirm that the correct DNA sequence is present in that particular clone.
Genetic engineering is not limited to bacteria. The genomes of other microorganisms, plants, and animals can be altered in a similar way. Many agricultural plants, such as corn, canola, or soybeans have already been genetically engineered to improve their performance. Another application of GMOs is in the pharmaceutical industry. Today, many medicines, such as insulin for diabetes or certain antibodies, are produced by genetically modified bacteria or plants. Scientists are also looking into its use in the production of other commercially valuable products, such as spider silk, biofuels, and vaccines.
In this project, you will create your own genetically modified bacteria. Specifically, you will make Escherichia coli bacteria glow by introducing a green fluorescent gene (gfp) into their DNA. GFP originates from a jellyfish and it is often used in biotechnology to mark certain cells, or as a reporter gene that indicates if a cell expresses a certain protein. In this experiment, you will transform a plasmid that contains the gfp gene into Escherichia coli cells. Once the plasmid has been successfully taken up by the E. coli cells, they will fluoresce under UV light. During your experiment, you will vary the transformation conditions to determine how that changes the cells' ability to take up the GFP plasmid. The transformation efficiency will tell you how efficiently your cells took up the plasmid. The transformation efficiency can be determined by the number of successful transformants (glowing bacteria cells or colonies on the selective plate) generated per microgram of plasmid DNA used for the transformation and spread on the selective agar plate. How many glowing bacteria cells do you think you will have on your plates?
Terms and Concepts
- DNA
- Genetic engineering
- Gene
- Transcription
- Protein
- Translation
- Genetically modified organism (GMO)
- Host cell
- Recombinant DNA
- Molecular cloning
- Restriction enzymes
- DNA ligase
- Vector
- Plasmids
- Transformation
- Agar plates
- Escherichia coli
- Green fluorescent gene (gfp)
- Transformation efficiency
Questions
- What is the role of plasmids in molecular cloning?
- How can you increase or decrease the transformation efficiency of a reaction?
- How are genetically modified organisms (GMOs) used today?
Bibliography
- New England BioLabs. (n.d.). Foundations of Molecular Cloning - Past, Present and Future. Retrieved August 28, 2020.
- Phillips, T. (2008). Genetically modified organisms (GMOs): Transgenic crops and recombinant DNA technology. Nature Education 1(1):213
- Millipore Sigma (n.d.). Bacterial Transformation. Retrieved August 28, 2020.
Materials and Equipment
- Genetic Designer Starter Kit - Glowing Jellyfish Bacteria, available from The ODIN. To get 10% off the purchase price of the kit, use the coupon code "buddies" at checkout. Read the Procedure to see if you want one or two kits. The kit includes:
- LB agar (1)
- LB Kan agar (Kan [100 μg/ml]) (1)
- 250 mL glass bottle for pouring plates (1)
- Disposable transfer pipette (9)
- Petri dishes (7)
- Inoculation loops (5)
- Pairs of nitrile gloves in plastic bag (5)
- Bag of microcentrifuge tubes (1)
- 1.5-mL microcentrifuge tubes containing LB broth (5)
- 50-mL centrifuge tube for measuring liquid volume (1)
- 100 μL bacterial transformation buffer (25 mM CaCl2, 10% PEG 8000) (5)
- Orange UV filter sheet and blue light (1)
- Non-pathogenic E. coli bacteria freeze-dried tube (DH5α) (1)
- Sterile water tube (1)
- Jellyfish GFP plasmid freeze-dried tube (5)
- Pencil or pen
- Permanent marker
- Calculator
- Lab notebook
Disclaimer: Science Buddies participates in affiliate programs with Home Science Tools, Amazon.com, Carolina Biological, and Jameco Electronics. Proceeds from the affiliate programs help support Science Buddies, a 501(c)(3) public charity, and keep our resources free for everyone. Our top priority is student learning. If you have any comments (positive or negative) related to purchases you've made for science projects from recommendations on our site, please let us know. Write to us at scibuddy@sciencebuddies.org.
Experimental Procedure
Bacterial Safety
Bacteria are all around us in our daily lives and the vast majority of them are not harmful. However, for maximum safety, all bacterial cultures should always be treated as potential hazards. This means that proper handling, cleanup, and disposal are necessary. Below are a few important safety reminders.
- Keep your nose and mouth away from tubes, pipettes, or other tools that come in contact with bacterial cultures, in order to avoid ingesting or inhaling any bacteria.
- Make sure to wash your hands thoroughly after handling bacteria.
- Proper Disposal of Bacterial Cultures
- Bacterial cultures, plates, and disposables that are used to manipulate the bacteria should be soaked in a 10% bleach solution (1 part bleach to 9 parts water) for 1–2 hours.
- Use caution when handling the bleach, as it can ruin your clothes if spilled, and any disinfectant can be harmful if splashed in your eyes.
- After bleach treatment is completed, these items can be placed in your normal household garbage.
- Cleaning Your Work Area
- At the end of your experiment, use a disinfectant, such as 70% ethanol, a 10% bleach solution, or a commercial antibacterial kitchen/bath cleaning solution, to thoroughly clean any surfaces you have used.
- Be aware of the possible hazards of disinfectants and use them carefully.
For health and safety reasons, science fairs regulate what kinds of biological materials can be used in science fair projects. You should check with your science fair's Scientific Review Committee before starting this experiment to make sure your science fair project complies with all local rules. Many science fairs follow Regeneron International Science and Engineering Fair (ISEF) regulations. For more information, visit these Science Buddies pages: Project Involving Potentially Hazardous Biological Agents and Scientific Review Committee. You can also visit the webpage ISEF Rules & Guidelines directly.
The ODIN kit contains enough materials to do five experiments. It also comes with a kit manual that includes the instructions for how to do the experiment. You can also access the kit manual online. In this experiment, you will investigate how the transformation conditions affect the bacteria's capability of taking up the GFP plasmid into their cells.
Engineering the Bacteria
- When you receive the kit, make sure to store all the perishables in the refrigerator, as advised in the kit manual.
- Follow the kit instructions to make the agar plates.
- Continue to make the competent cells, as instructed in the kit manual.
- You will change the transformation conditions to test how this affects the transformation efficiency. The changes will happen during the heat shock step.
- Follow the first two steps of the transformation protocol (adding the water to the freeze-dried plasmid and mixing it with the competent cell mixture, as well as incubating the transformation mix in the refrigerator for 30 minutes).
- In the next step, you will test different heat shock conditions. Specifically, you will vary how long the cells will be heat shocked at 42°C. As in traditional science projects, you should repeat your experiments to make sure your results are replicable. In this experiment, you will test two different heat shock conditions and do these experiments in duplicate. If you have a second kit available, you can also test three different heat shock conditions and do triplicates of these. Depending on your resources, choose one testing scenario for your experiment, based on Table 1.
Heat Shock Time
(seconds [s])1 Kit Available 2 Kits Available 15 x Triplicates 30 Duplicates Triplicates 60 Duplicates Triplicates
- Incubate the tube for 15 s in 42°C water (only if you have two kits available).
- Incubate the tube for 30 s in 42°C water (as advised in the kit).
- Incubate the tube for 60 s in 42°C water.
- We recommend you do all replicate experiments in parallel so all the conditions remain the same.
- Then continue with the preparation of the LB recovery media, as described in step 4 in the transformation protocol, and finish the experiment by following the kit instructions to the end. Note: Make sure to write the transformation conditions on your plates before you spread your bacteria.
Analyzing Your Results
- Count how many glowing cell colonies you have on each plate. A good way to do this is to mark each counted colony with a dot, so you do not count them twice. Write down the total number of colony-forming units (cfu) for each of your plates.
- Then calculate the average number of glowing colonies for each transformation condition.
- If you can, take a picture of all your plates for your display board.
- Calculate the transformation efficiencies for each transformation condition. Review the Technical Note for an example calculation.
- Determine the amount of GFP plasmid DNA in the bacteria spread on the LB/Kan agar plates.
Note: The concentration of the GFP plasmid in the kit is 20 ng/μL.- Determine the total amount of GFP plasmid DNA in the transformation mix.
- Determine the fraction of GFP plasmid DNA that actually got spread onto the LB/Kan agar plates.
- Calculate the total amount of GFP plasmid DNA spread on the LB/Kan agar plates.
- Use the average number of colonies on your plates and the calculated amount of GFP plasmid DNA spread on the plates to calculate the transformation efficiencies for each transformation condition.
- Compare the different transformation efficiencies. How do they differ? Which transformation condition resulted in the highest or lowest transformation efficiency? What do your results tell you about the state of the competent cells in the transformation mix? Can you explain why one transformation condition worked better than the other?
Technical Note: How to calculate the transformation efficiency - Example Calculation
To calculate the total amount of plasmid DNA that you plated, you need to know the original concentration of your DNA plasmid and the volume of plasmid that you add to the transformation mix. You also have to take into account that you add LB medium to the transformation mix to recover the cells and only use a fraction of that volume to actually spread on the agar plates.
An example calculation is shown below.
In an experiment, 10 μl of plasmid at a concentration of 0.08 μg/μl is added to 100 μl of transformation mix. After the transformation, 400 μl of LB medium are added to the competent cells for recovery. Finally, 100 μl of the recovered cells are spread onto the agar plate. The next day, 190 green fluorescent cell colonies are counted on the plate.
The total amount of DNA used in the experiment is:
The fraction of DNA used for plating is:
(Note: In the test tube are 10 μl plasmid, 100 μl transformation mix, and 400 μl LB medium = 510 μl)
Based on that information, the total amount of DNA spread on the agar plate is:
The transformation efficiency can now be calculated as:

Ask an Expert
Global Connections
The United Nations Sustainable Development Goals (UNSDGs) are a blueprint to achieve a better and more sustainable future for all.
Variations
- Find out how the heat shock temperature affects the transformation efficiency. Repeat the experiment, but this time keep the heat shock time constant at 30 s and vary the temperature of the water. Does increasing or decreasing the water temperature have an effect on the transformation efficiency?
- How important is the cell recovery phase after the heat shock treatment of the cells? Find out how the transformation efficiency changes based on how long you recover the cells after the heat shock.
- Despite the potential benefits and promises of genetic engineering, there are also concerns and risks associated with GMOs that need to be considered. Do some research and find arguments for and against the use of GMOs.
- In this project, you have engineered the bacteria Escherichia coli. You can do the same experiment with eukaryotes, such as yeast, in the Genetically Engineer Yeast to be Fluorescent Science Buddies project.
- Genetic engineering has led to gene editing, which is a technology that allows us to change and edit genes within organisms at precise locations. CRISPR is an emerging technology that has become an important tool for gene editing. In the CRISPR Gene Editing of Escherichia coli Science Buddies project, you can do your own gene editing experiment using CRISPR.
Careers
If you like this project, you might enjoy exploring these related careers:
Related Links
- Science Fair Project Guide
- Other Ideas Like This
- Biotechnology Project Ideas
- Genetic Engineering Project Ideas
- My Favorites